Lucy Letby: insulin and C-Peptide in preterm neonates
- drellenstorm
- Mar 11
- 10 min read
Updated: Mar 16
Please note: the information provided below represents the current understanding and opinion of Dr Ellen Storm on the 10th March 2025, based on the information available to her at the time, and does not represent the viewpoints of any organisations with which she may be associated. Other specialists may wish to comment on and/or clarify some of the points made below. Dr Storm reserves the right to update her opinion based on the provision of further information in the future.
Background:
The following has been compiled in response to the following table, which was posted on Facebook in March 2025:
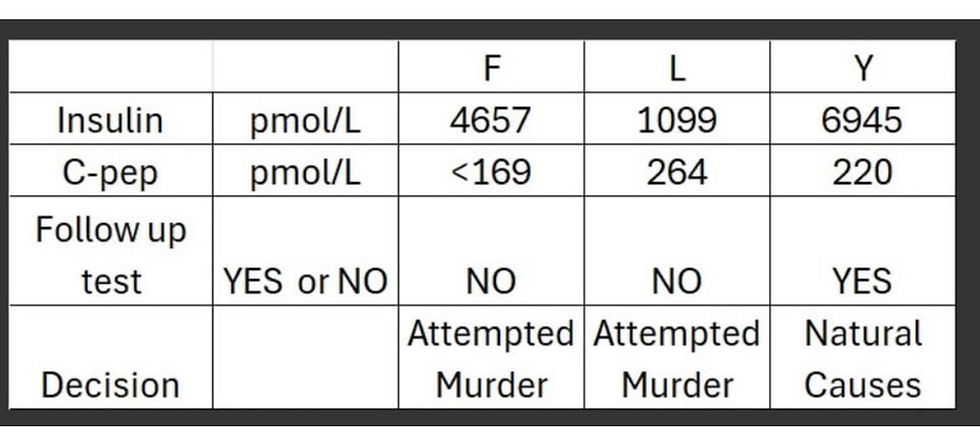
A brief literature search was carried out via OpenAthens using the search terms ‘c-peptide’ and ‘neonate’. A full literature search is recommended to fully scope the data available on this subject.
Question:
What is the likelihood that the observed values of C-peptide and insulin (pmol/L) in Baby F (<169/4657), Baby L (264/1099) and Baby Y (220/6945) were all ‘false positives’, i.e. that the true values were actually within the normal, or ‘reference’, range?
Answer:
Assuming the correct functioning of the assay, there will always be a degree of sampling variability within a range (for example, three different samples taken from the same patient at the same time and run on the same machine will likely yield different values within a certain range. This range should be known for any population for whom an assay is to be applied as a screening or diagnostic test for any particular condition (in this case congenital hyperinsulinism), and should be relatively narrow.
A narrow range allows for greater sensitivity and specificity of the test. Greater sensitivity means it is more likely to accurately detect the condition if it is there, whereas a wider range will miss some true positives (resulting in more false negatives). Greater specificity means it is more likely to accurately exclude the condition if it is not there, whereas a wider range will miss some true negatives (resulting in more false positives).
The range of sampling variability is different from the population reference range, which should also be known for any assay that is to be used to screen for or diagnose a particular condition in a particular population. The population reference range is derived by taking samples from a number of unaffected individuals who share similar characteristics (e.g. birth weight and gestational and postnatal age). The reference range is usually two standard deviations[1] about the mean, meaning that 5% of results in a population of healthy individuals would be expected to lie outside that range (Lab Tests Online UK, 2021).
For insulin, for the test to accurately diagnose congenital hyperinsulinism (CHI), reference ranges also need to take into account blood sugar at the time of the test (i.e. they should tell us what level of insulin might be expected for different blood sugar levels in infants who do not have the condition). Under normal physiological circumstances, it would be expected for insulin levels to be lower with lower blood sugars, and to rise as blood sugar rises, as the function of insulin is to move glucose from the circulation into the cells. The combination of high insulin and low blood sugar is suggestive of CHI.
More extremely aberrant results might be expected to be seen as a result of assay malfunction or incorrect calibration. Calibration data for the assay at the time of testing would be necessary to confirm its reliability.
If it is true that no data is available for the range of sampling variability, the sensitivity, specificity, and positive and negative predictive values of the tests, or the population reference ranges for both parameters, for populations of preterm infants of different birth weights and gestational ages, and at different levels of blood glucose, then it is not possible to answer this question.
Conclusion:
If it is true that no data is available for the range of sampling variability, the sensitivity, specificity, and positive and negative predictive values of the tests, or the population reference ranges for both parameters, for populations of preterm infants of different birth weights and gestational ages, then this test should not be being used in a clinical setting for these populations of infants, as it is not possible to correctly interpret the results. If it is used in the clinical setting, it should be understood to be a guide only, and interpretation should be with caution and with the caveat that these reference values and ranges have not yet been established.
Question:
How common is congenital hyperinsulinism (CHI) in premature neonates?
Answer:
According to Congenital Hyperinsulinism International (n.d.), CHI affects 1 in 28,000 births, and is ‘the most frequent cause of severe, persistent hypoglycemia in newborn babies and children’.
According to the CHI team at Alder Hey (2022): ‘Hypoglycaemia due to CHI is relatively rare but potentially a serious condition occurring soon after birth. The estimated incidence of CHI is 1 in every 40,000 - 50,000 children although it is likely that the incidence is higher’.
According to the CHI team at Alder Hey (2022): ‘There are different forms of CHI. These can often be distinguished by the length of treatment required and the infant’s response to medical management. Some forms will resolve and are considered transient. Others arise from genetic defects and persist for life. These genetic forms of CHI do not go away, but in some cases, may become easier to treat as the child gets older’.
According to the CHI team at Alder Hey (2022): ‘At present, there are nine known genetic causes of CHI, which can be inherited in an autosomal recessive (one mutation in the gene inherited from each parent, neither of whom is affected) or dominant manner (a mutation in a single copy of the gene). Abnormalities in the genes ABCC8 and KCNJ11 are the most common cause of severe CHI’.
According to the CHI team at Alder Hey (2022): ‘Transient CHI means that the increased insulin production is only present for a short duration and is found in conditions such as:
Intrauterine growth retardation (babies with low birth weight)
Prematurity
Infants of diabetic mother
Infants with perinatal asphyxia (issues with low oxygen at birth)
Transient hyperinsulinism can occur in infants with no predisposing factors, the mechanisms causing this is currently unclear. Some of the transient forms of CHI will need treatment with medications for a few weeks to a few months; but generally, will be able to come off treatment at a later date. This will be assessed by a fasting study while off all medications to prove that the hyperinsulinism has completely resolved’.
It is not clear from these sources whether or not the incidence figures given above include all cases of transient CHI, or only refer to genetic CHI persisting into childhood. Therefore, the incidence including all cases of transient CHI may be higher.
Conclusion:
It is not possible to be sure of the total incidence of CHI, including both genetic and all transient neonatal cases, based on the information available at the time of writing. Further information is required in order to answer this question: specifically, whether or not any studies have been done specifically to determine the incidence of hyperinsulinaemia in premature neonates at different gestational ages. Failing that, as a more general guide, how frequently hyperinsulinism is generally found and treated in an average level 2 or 3 neonatal unit might be helpful to know. Therefore, it is not currently possible to draw any conclusions as to whether or not three cases of CHI in one unit might be regarded as exceptional.
Question:
How might we account for the observed values of C-peptide and insulin (pmol/L) in Baby F (<169/4657), Baby L (264/1099) and Baby Y (220/6945), if a) the obtained values are true (i.e. they accurately reflect the true serum values at the time of sampling), and b) none of the babies received exogenous insulin immediately prior to that sampling?
Answer:
Pro-insulin is produced in the beta cells of the pancreas.
Pro-insulin is split into insulin and C-peptide, giving an initial 1:1 ratio.
Standard teaching advises that endogenously produced hyperinsulinaemia results in approximately equimolar concentrations of insulin and C-peptide, whereas exogenous insulin administration produces a high level of insulin and a low level of C-peptide (since C-peptide is not present in synthetic human insulin analogues produced by recombinant DNA technology).
However, this teaching is incorrect in relation to babies and children.
Halliday (1986) reported on C-peptide levels in a case of transient neonatal diabetes starting on the second day of life, stating that: ‘C-peptide levels were measured to aid in the assessment of insulin treatment. Very low levels were found for the first 5 months of life (less than 0.06 nmol/L [60 pmol/L])’.
In 1981 Knip and Akerblom reported their study of the insulin and C-peptide concentrations in 76 infants and children divided into five groups:
Group 1 – cord blood was taken for 20 term infants
Group 2 – blood was taken from 12 healthy term infants between 36 and 60 hours of age after four hours of fasting
Group 3 – blood was taken from 12 normal-weight infants aged 0.5 to 12 months
Group 4 – blood was taken from 12 normal weight children aged 1.5 to 6.0 years who were fasted prior to elective surgery
Group 5 – blood was taken from 20 normal-weight children aged 6.9 to 15.1 years who were fasted prior to elective surgery
Insulin and C-peptide were measured in nmol/L and mU/L respectively.
The ‘molar ratio’ was then calculated, which is the ratio of C-peptide to insulin.
The cited mean molar ratios were:
Group 1 = 3.15
Group 2 = 2.95
Group 3 = 5.28
Group 4 = 4.90
Group 5 = 5.23
For better comparison, Table 1 below presents the molar ratios in pmol/L (values converted using Insulin conversion calculator to pmol/L, µIU/mL, mIU/L units. Online converter from conventional to SI units | UNITSLAB.COM ) for the groups in this study and for babies F, L and Y. For correct interpretation it should be remembered that the study participants were fasting at the time that blood was drawn, and this would be expected to result in relatively low insulin values, whereas the babies F, L and Y were (presumably?) receiving intravenous infusions of glucose (+/- bolus doses), which would be expected to increase insulin secretion:
Table 1:
| CP nmol/L | CP pmol/L | IRI mU/L | IRI pmol/L | MR study | MR pmol/L |
Group 1 | 0.242 | 242 | 13.8 | 95.8 | 3.15 | 2.53 |
Group 2 | 0.122 | 122 | 7.0 | 48.6 | 2.95 | 2.51 |
Group 3 | 0.225 | 225 | 7.4 | 51.4 | 5.28 | 4.38 |
Group 4 | 0.178 | 178 | 6.2 | 43.1 | 4.90 | 4.13 |
Group 5 | 0.414 | 414 | 13.3 | 92.4 | 5.23 | 4.48 |
Baby F |
| 169 |
| 4657 |
| 0.04 |
Baby L |
| 264 |
| 1099 |
| 0.24 |
Baby Y |
| 220 |
| 6945 |
| 0.03 |
The authors note that: ‘The molar ratio of C-peptide to IRI was lower in cord blood and neonates than in the older age groups’ and that ‘The fasting plasma C-peptide correlated in 56 children (cord blood excluded) to the age of the children’.
The authors note that: ‘The molar ratio of C-peptide to IRI[2] is determined by the balance between the rates of removal for C-peptide and insulin. The kidney is the major site for C-peptide metabolism, whereas the liver is the primary organ of insulin degradation. The low molar ratio of C-peptide to IRI in 2-day-old infants could be explained by a diminished hepatic extraction of insulin in this age group. The “relative” excess of insulin in peripheral circulation in neonates, being a consequence of decreased hepatic degradation, could also explain why plasma IRI concentration correlates less well than C-peptide with the age of the children’.
The authors conclude that: ‘the hepatic extraction of insulin seems to be diminished in neonates, leading to a low molar ratio of C-peptide to insulin in the peripheral circulation’.
Conclusion:
It is notable that in none of the cases tabled above was the molar ratio found to be 1. The molar ratio of >1 in the healthy fasting subjects indicates a rate of insulin clearance higher than the rate of C-peptide clearance. This might be reasonable to expect in healthy subjects whose liver and kidneys are functioning normally. The short half-life and short duration of action of synthetic insulin is a function of its rapid first-pass metabolism by the liver. According to the British National Formulary (n.d.) intravenous soluble insulin ‘has a short half-life of only a few minutes and its onset of action is instantaneous’. Therefore, in healthy subjects, insulin is generally metabolised more rapidly than C-peptide.
Knip and Akerblom (1981) identified reduced hepatic function in healthy neonates as a potential explanation for their observed differences, but did not identify increased renal excretion. If insulin and C-peptide are produced by the pancreas in equimolar amounts, the only physiological explanation for a molar ratio other than 1 is differential rates of breakdown and/or excretion. A molar ratio <1 (C-peptide less than insulin) can only be the result of the rate of renal excretion of C-peptide being higher than the rate of hepatic metabolism of insulin. Might this conceivably occur in sick premature neonates?
C-peptide, or the urinary C-peptide to creatinine ratio (UCPCR), is commonly measured in the urine of insulin-dependent diabetics to quantify their levels of endogenous insulin production. Therefore, C-peptide is not only metabolised by the kidney, but also renally excreted in the urine. Loss of protein in the urine (proteinuria) is a problem that affects premature infants due to the immaturity of their kidneys.
Trigolet et al (2023) tested 1140 urine samples from 190 very preterm (<31 weeks) infants in the first month of life for urinary protein to creatinine ratio. They found that ‘Preterm infants have physiological proteinuria and values of urine protein to creatinine ratio (UPr/Cr) are higher compared to full-term infants during the first week of life’. They go on to report that ‘At the multivariate analysis, lower gestational age (GA) and increasing postnatal age were the only variables significantly associated with higher UPr/Cr values (p < 0.001). There was wide intra- and interindividual variability in UPr/Cr, especially in infants with higher GA and clinical stability’.
From this we must conclude that it is physiologically plausible that sick preterm babies who have impaired hepatic and/or renal function (specifically proteinuria) may have a molar ratio of <1. However, more research is needed to accurately delineate this relationship, which is currently poorly understood.
References:
ALDER HEY, 2022. Congenital Hyperinsulinism (CHI): Information for parents (online). Available from: https://www.alderhey.nhs.uk/wp-content/uploads/2023/06/Congenital-Hyperinsulinism-CHI-Leaflet-PIAG-32.pdf (Accessed 11 March 2025).
BRITISH NATIONAL FORMULARY, n.d.. Insulin (online). Available from: Insulin | Treatment summaries | BNF | NICE (accessed 11 March 2025).
CONGENITAL HYPERINSULINISM INTERNATIONAL, n.d.. About Congenital Hyperinsulinism (online). Available from: https://congenitalhi.org/congenital-hyperinsulinism/ (Accessed 11 March 2025).
HALLIDAY, H.L., et al., 1986. C-peptide levels in transient neonatal diabetes. Diabet Med. 3(1):80-1.
LAB TESTS ONLINE UK, 2021. Reference Ranges & What They Mean (online). Available from: https://labtestsonline.org.uk/articles/laboratory-test-reference-ranges (Accessed 11 March 2025).
TRIGOLET, M., BONSANTE, F., GUIGNARD, J-P., GOUYON, J-B., IACOBELLI, S., 2023. Urinary protein to creatinine ratio during the first month of life in very preterm infants – a prospective cohort study (PROTIPREMA). Pediatr Nephrol. 38 (3), pp.721-727.
[1] Standard deviation (SD) is a statistical measure of the spread of a group of data points about their mean, calculated as the square root of the variance. A lower SD indicates that the points are more tightly clustered around the mean, whereas a higher SD indicates that they are more spread out.
[2] IRI – Immunoreactive insulin
コメント